Greening Hydrogen: Challenges, Innovations, and Opportunities
Technological advancements, policy interventions, and market shifts are shaping the future of green hydrogen in the global race to decarbonize. Here are four key points about its current state and the steps needed to unlock its full potential.
Like many in the green energy space, LONGi Green Energy Technology Company is ready to figure out whether green hydrogen—a potential source of renewable energy—can actually be a viable solution in society’s race to decarbonize.
In recent years, top decision makers at LONGi, a massively successful solar power company founded in 2000, have seen the company’s profitability slashed by plunging solar power prices and have determined that the firm’s hyperfocus on solar modules should expand to include a diversification into new growth areas. As such, in 2021, LONGi launched LONGi Hydrogen Energy Technology Company to manufacture green hydrogen equipment. The new arm’s first product was an alkaline water electrolyzer, which uses electricity to split water (H2O) into hydrogen and oxygen in a process called electrolysis. When the process is powered by renewables, it yields green hydrogen, an energy source devoid of carbon emissions.
But it is early days for green hydrogen. By 2024, LONGi was the world’s largest manufacturer of electrolyzers, and yet its green hydrogen equipment business generated a scant 1% of the company’s total revenues. In fact, green hydrogen accounts for less than 1% of all hydrogen produced, over 98% of which is still derived from natural gas. Overall, hydrogen represents a tiny slice of the world’s energy market, accounting for less than 1% of the global energy mix.
And yet, plenty of energy experts agree that green hydrogen can’t be discounted. Hydrogen has been called the Swiss Army knife of decarbonization because of its adaptability to a range of high-emitting areas across industry, power, heat, and some forms of transportation, such as marine fuels. It is also true, of course, that while Swiss Army knives can do lots of things, they aren’t exactly known for doing any one function particularly well.
Swiss Army knives shine, when there is a lack of alternatives—as may well be the case with green hydrogen. The business case will work for applications, where there are few viable alternatives.
Two of the biggest barriers to the expansion of green hydrogen have been its relatively high price—due mostly to the cost of electrolyzers and the renewable energy to power them—as well as infrastructure and compatibility problems with current power systems. What’s clear, and what adds to the uncertainty surrounding the space, is that the scale-up of green hydrogen will be heavily dependent on policy support.
Thanks to its relevance to many otherwise hard-to-abate sectors, global demand for hydrogen is projected to increase significantly in the coming decades, from 125 million tonnes (Mt) per year in 2030 to over 500 Mt by 2050. If demand does indeed track this steep upward path, it will require a dramatic scale-up in production—and oil majors and utilities are taking note. Saudi Aramco acquired a 50% equity stake in Blue Hydrogen Industrial Gases Company in 2024; the year prior, the utility company NextEra Energy announced a plan for full decarbonization that includes converting 16,000 megawatts of natural gas generating units to run on green hydrogen. The year before that, British oil and gas company BP acquired a $36 billion stake in what is now the Australian Renewable Energy Hub, which will produce 1.6 Mt of green hydrogen per year upon completion.
Then there is low-carbon steel. Stegra, formerly known as H2 Green Steel, is expected to complete the world’s first full-scale low-carbon steel plant by 2026. Since Stegra’s announcement, over a dozen other companies have announced their own intentions to produce over 40 Mt of low-carbon steel by 2030 in Europe alone.
Whether or not green hydrogen’s rise will meet these projections and ultimately play a central role in helping the world achieve decarbonization will depend upon more than whether existing technology can scale up. Much more work remains to be done in the space to develop new technologies, address infrastructure and compatibility issues, and formulate policies that can effectively jump-start a flow of hydrogen energy that becomes progressively greener as the years pass—not less so.
Read on for four key points about the current state of green hydrogen development and where it needs to go from here.
Key Point No. 1: Incorporating hydrogen into the energy system is not a fanciful, futuristic idea—it’s already being done. Still, plenty about hydrogen and its use needs to change.
Today, hydrogen is commonly used as an input in petroleum refining, where it acts to break apart complex hydrocarbons and remove impurities. Most of the hydrogen used for this purpose, and others, is produced from natural gas, a process that releases carbon dioxide.
Hydrogen made this way—from natural gas, with CO2 as a byproduct—is commonly known as grey hydrogen, which describes nearly all hydrogen production today, representing over 98% of the current hydrogen market.
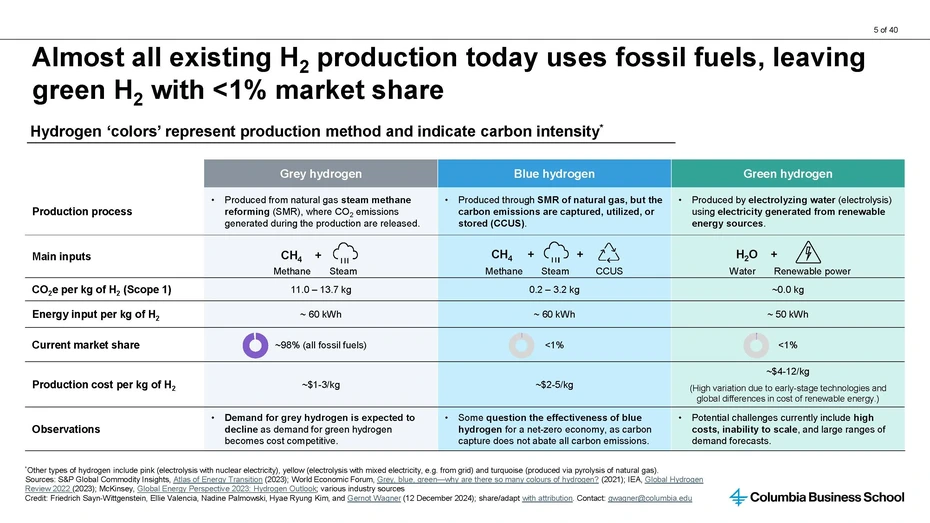
Blue hydrogen, though it’s produced in the same way, earns a different color-coded designation because the carbon dioxide emitted through its production is captured and stored or utilized. Green hydrogen, on the other hand, is produced without using natural gas, whereby water is electrolyzed to split hydrogen from oxygen—releasing no carbon in the process.
However, while the benefits of green hydrogen for the climate are obvious, the economics are less so.
Green hydrogen is by far the most expensive of these variations to produce. Grey and blue hydrogen benefit from the relatively lower costs of fossil fuels, with grey hydrogen averaging between $1 and $3 per kilogram and blue running between $2 and $5 per kg (with costs inflated by the costs of carbon capture and utilization or storage, or CCUS). In comparison, truly green hydrogen commands at least double those prices: an average of between $4 and as much as $12 per kg, due to the costs of electrolyzer technology and renewable energy prices. (Powering electrolyzers with electricity from the grid is cheaper yet also not truly green, as the electricity mix on the grid typically is not.)
The upshot is, if green hydrogen is to ramp up quickly enough to become a significantly larger share of hydrogen production, its cost must come down. An open question remains as to how innovations in electrolyzer technology and renewable energy deployment, alongside strategic policy interventions, can bring this about—and whether the market is sufficiently motivated to figure it out.
Key Point No. 2: Distinct technology pathways for green hydrogen are emerging—and each brings its own challenges and upsides.
Given that electrolyzers are the technology at the heart of green hydrogen production, it’s no surprise that the particularities of different electrolyzers have distinct implications for its ramp-up.
The alkaline water electrolyzer (AWE) currently dominates the market, with a roughly 60% share. AWEs achieve electrolysis using nickel electrodes, making them cost effective and durable. They are also the type currently manufactured by LONGi. At present, these electrolyzers are by far the least expensive on the market. However, they come with a major drawback: They require continuous electricity supplies for optimal production, ruling out dependence on the most widely available types of renewable energy.
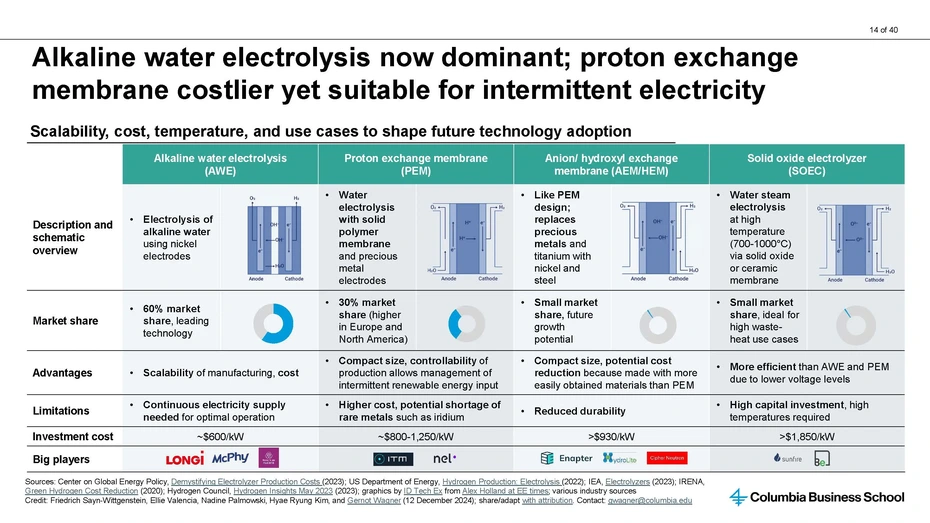
Proton exchange membrane (PEM) electrolyzers, on the other hand, do better with intermittent renewable energy sources like solar and wind energy. Thanks to this advantage, PEM electrolyzers command about 30% market share, despite being far more expensive than their AWE counterparts.
But PEM electrolyzers present another hitch: They perform water electrolysis with solid polymer membranes and precious metal electrodes, and some of the metals required, like iridium, are in short supply. If PEM is to become a more promising source of electrolysis, new research and innovation will be required to tweak the technology and bring down its costs.
A third form of electrolyzer, anion/hydroxyl exchange membrane (AEM/HEM), accounts for a much smaller slice of the market, though many believe it’s a promising direction because the required materials—typically nickel, instead of titanium for PEM—are more common than those required by PEM. Unfortunately, this variation also comes with lower durability than other electrolyzer technologies, which means that, once again, more innovation is needed.
A fourth type is the solid oxide electrolyzer (SOEC), currently a tiny slice of the market, in part because of high capital investment costs and the high temperatures required. It is particularly viable for specific use cases such as industrial processes with high amounts of excess heat.
The distinctions between these four electrolyzer technologies point to manifold possibilities when it comes to separating hydrogen from oxygen—and also the often-devilish details that will need to be worked out, no matter which path is chosen.
Key Point No. 3: Hydrogen promises to help decarbonize some otherwise hard-to-abate sectors, including steel and shipping.
The largest driver of current low-carbon hydrogen demand is the chemicals industry. Hydrogen and nitrogen are the two key ingredients needed to produce ammonia via the Haber-Bosch process. Future growth for (green) hydrogen is expected to come from other industrial sectors like steel and transport, especially shipping.
Some also see green hydrogen as key in decarbonizing long-haul road transport and aviation. Hydrogen fuel cell electric vehicles may prove superior to full electrification for long-haul trucking thanks to faster refueling, better ranges, and the fuel’s lighter weight. However, fuel cell technology will need to compete with ever-cheaper battery technologies and a concerted push for full-on electrification of road transport.
In the shipping sector, hydrogen in the form of liquid hydrogen or synthetic fuels can fuel ships directly, though the fuel’s lower energy density in this form makes transport on ships more difficult and requires carriers, like ammonia.
Low-carbon hydrogen also shows some promise in aviation, with Rolls Royce and Airbus experimenting with hydrogen engines and planes. One key challenge preventing rapid uptake in this space, as in so many others, remains high costs.
Key Point No. 4: It’s crucial that policymakers around the world clearly and thoughtfully address a pressing question: What, exactly, constitutes green hydrogen?
Given green hydrogen’s market hurdles, it’s fair to say the fuel’s success currently depends on well-crafted political subsidies, tax credits, and financial initiatives.
Many governments are acting accordingly: Over 30 jurisdictions, representing roughly 80% of energy-related carbon emission, have adopted national hydrogen strategies, with the European Union and the United States currently in the lead. Both sets of governments are backing research, development, and demonstration projects, offering grants and tax incentives, and establishing standards for large-scale hydrogen projects.
This last piece—standard setting—is crucial: What constitutes green hydrogen and is therefore eligible for generous tax credits and other support?
Three often-mentioned criteria are that green hydrogen must prove itself to be new (meaning brand-new installations of wind, power, or other renewables must be powering the projects); nearby (ensuring hydrogen projects are close to their suppliers of clean energy); and matched quickly with demand (hydrogen should be produced in line with when its renewable electricity is produced—when the sun is shining or the wind is blowing—so it’s not drawing from dirtier sources of power).
Getting these criteria right, including tax credits and other government subsidies more broadly, will be key in efforts to help the green hydrogen industry achieve its decarbonization potential.
Greening Hydrogen: Challenges, Innovations, and Opportunities