Two Bookends For The Reincarnation Of Hydrogen—ExxonMobil And Verdagy
Why Hydrogen?
The most prominent use of liquid hydrogen is for hard-to-abate heavy industries such as manufacturing of chemicals and fertilizer, cement and metals such as steel and aluminum. The hard-to-abate list also includes fuels for aviation, shipping, and long-haul trucking fuels. All these industries are difficult to run on electricity, because they need fuels with high energy density such as coal, coke, diesel, or kerosene. But all these fuels emit high volumes of greenhouse gases (GHG).
An obvious replacement is liquid hydrogen which has high energy density but essentially zero emissions. Big oil companies are well-suited to hydrogen generation because of their vast experience in natural gas, and they have deep pockets. A prime example is ExxonMobil.
But there are smaller hydrogen applications that could be called light industry: transport by buses and cars, cargo cranes, heating for buildings, and cooking. Replacing natural gas in power plants is also feasible. Large-scale applications of hydrogen in heavy industries are limited to 10-15% of decarbonization needed across the world, according to Rystad Energy. But how large might be the contribution of small-scale supplies of hydrogen? One such is BayoTech out of Albuquerque, New Mexico, who have successfully generated and delivered hydrogen to fuel-cell buses that service both state and school. Using biomethane as feedstock, their hydrogen is lower in carbon than standard-methane feedstock in blue hydrogen.
Blue Or Green Hydrogen?
Cost is an enduring question in regard to hydrogen generation, and it’s a bipolar situation. First, blue hydrogen accounts for 95% of hydrogen generation, partly because of its lower cost ($2-5/kg). Under steam heat, the process reforms methane gas into hydrogen and carbon dioxide, CO2. Methane is plentiful as it’s the primary component of natural gas, so it comes easy to oil and gas companies. But the problem is the CO2 has to be sequestered if the overall process is to be labeled “low-carbon”. Carbon capture and storage (CCS) generally implies storage of CO2 in deep underground formations such as old oil and gas fields, or saline layers. The cost includes CO2 disposal. Second, green hydrogen is more direct as it separates water into hydrogen and oxygen by electrolysis. It’s harder to do and is more expensive ($4.5-12/kg). But its cleaner in emissions, especially if wind or solar renewables are used to drive the electrolytic cells, in which case the process is “zero-carbon”. The U.S. Department of Energy (DOE) has set a goal of $2/kg which is associated with seven major hydrogen hubs set up within the past year, and funded to the tune of about $1 billion each. Some projects are blue hydrogen while others are green hydrogen
Let’s take a look at an example of a small-scale green hydrogen startup in 2021 (about 100 employees total) and a large-scale blue hydrogen project that was built in 1919 (62,000 employees total). Both projects are in the U.S. It’s insightful to compare the pros and cons of each project.
Green Hydrogen On Small Scale—Verdagy
Verdagy Inc. is a small company that runs a factory in Silicon Valley and a hydrogen plant in Moss Landing north of Monterrey, California. The latter is a 2 MegaWatt (MW) alkaline green hydrogen system. To be clear, Verdagy sells hydrogen electrolysis systems but does not sell hydrogen.
In the Verdagy system, the electrolyte is liquid, usually potassium or sodium hydroxide, with two electrodes separated by a membrane.
This contrasts with the PEM electrolyzer, standing for polymer electrolyte membrane (PEM), where the electrolyte is a solid plastic material. Another difference: in the Verdagy device, cells can be refurbished rather than replaced as in stack replacement in a PEM device, and this amounts to substantial cost savings.
According to Claudia Chow, a Verdagy company rep, the Moss Landing plant has been in operation for 12,000 hours over a period of about 3 years, which is equivalent to 1,500 eight-hour days. During this time, the plant has worked on reducing their capital costs and operating costs. They are creating a scalable design for manufacture that is on track to reach the DOE’s goal of $2/kg of levelized cost by 2026.
The company also claims their commercial process is efficient and highly reliable. This has been an unwavering problem with green hydrogen electrolyzers because they have been seriously inefficient.
Verdagy has entered into collaborations with other manufacturers and renewable energy companies. They are focused on generating green hydrogen with three general applications. First, as a feedstock in refining, petrochemicals, ammonia, and fertilizer, Second, fuel for aviation and marine transport. Third, factories that make steel, aluminum, and cement.
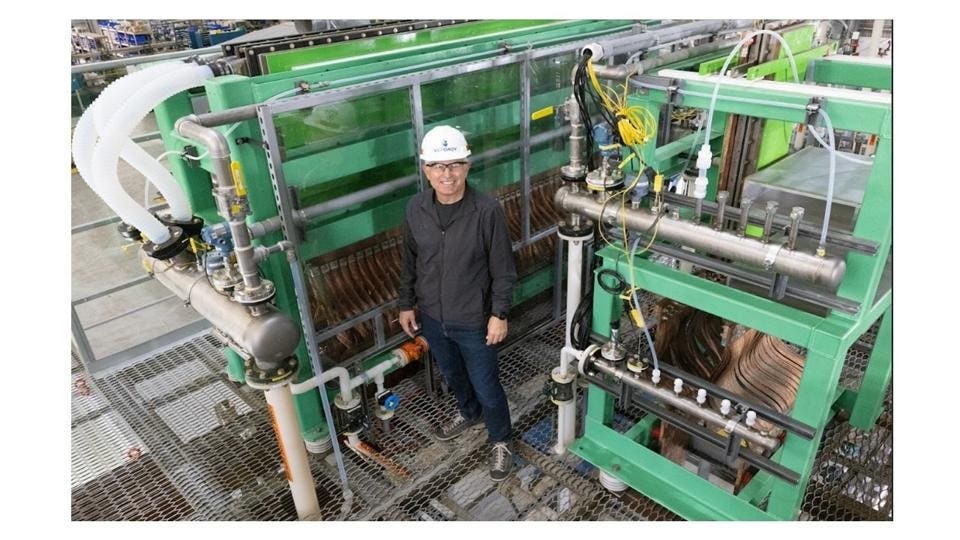
Marty Neese, CEO of Verdagy, Inc.
Verdagy
A Hydrogen Hub For California
In recent news, California has been awarded its very own Hydrogen Hub to the tune of $1.2 billion, which will be expanded by private investments of $11 billon. There will be 37 sub-projects, unnamed as yet, across the state. The kicker is, to meet the state’s climate goals, hydrogen generation in California will have to expand from about 7 million tons per annum to 71 million tons per annum by 2045. The state claims that 220,000 jobs will be created by the Hydrogen Hub, and almost $3 billion will be saved every year by avoiding diesel-related health issues.
It's hard to see Verdagy not being involved in the California hydrogen hub, which is specifically oriented toward green hydrogen.
Blue Hydrogen On Large Scale—ExxonMobil
ExxonMobil’s Baytown complex consists of a refinery, chemical plant, and olefins plant. Workforce is 2,000 permanent and 2,300 contract employees. The refinery close to Houston will be revamped to produce blue hydrogen plus ammonia (NH3), while the biproduct, CO₂, will be stored in a CCS project under the Gulf of Mexico. The hydrogen would be used for ExxonMobil’s olefin production plant, where carbon emissions could be reduced by 30%. Olefin is a synthetic fiber used in carpeting, wallpaper, and car interiors.
ExxonMobil are holding four keys to unlock hydrogen. First, 1 Bcfd of blue hydrogen and 1 million tons per year of ammonia will be produced in its Baytown refinery, while capturing more than 98% of the associated CO2 emissions. The company is already talking with potential customers to purchase surplus hydrogen and ammonia volumes in a 2027–2028 time-frame.
ExxonMobil and Air Liquide recently announced they will collaborate in production of low-carbon hydrogen and ammonia at ExxonMobil’s Baytown project. Using low-carbon electricity, Air Liquide will build four large air separation units to supply 9,000 metric tons of oxygen and up to 6,500 metric tons of nitrogen daily to the facility.
The final investment is subject to government policy, such as tax credits, plus necessary regulatory permits.
Second, seven hydrogen hub projects have been awarded hefty funds (about $1 billion each) from the DOE. One of these is called the HyVelocity Hub, centered along the US Gulf Coast, and organized by Chevron and several private company partners, including ExxonMobil and Mitsubishi (Palmer 2023h).
ExxonMobil will have its hands on critical elements of this hub project. First, the project would be leveraging on a network of forty-eight hydrogen-production centers (the largest in the world). Second, there will be a thousand miles of dedicated hydrogen pipelines along the Louisiana and Texas coasts. Third, ten thousand permanent jobs would likely result.
One technical goal is to solve the DOE’s challenge, called the Hydrogen Shot, of making 1 kg of hydrogen while emitting less than 2 kg of CO₂. Another goal is to reduce the cost of hydrogen by 80%—to $1/kg within ten years.
A third key that ExxonMobil holds is a plan to export blue ammonia from its Houston works to Japan. JERA is Japan’s largest power generation company, and they have signed an agreement that would export about half of the ammonia produced by the retreaded Baytown project. This is a big deal, although its conditional upon federal tax credits obtained by ExxonMobil.
Takeaways
The above illustrates a wide spectrum of ways and methods that hydrogen can be generated by the private sector. While the advances are exciting, it’s good to remember that hydrogen applications are basically for hard-to-abate heavy industries and these only occupy a small fraction of the world’s decarbonization goals (15%).
Even so, the U.S. administration is betting that the hydrogen effort will pay off. They are seeding the hydrogen market with truckloads of funding and hoping this will create jobs and demand for hydrogen, and yes, a substantial decline in GHG emissions. Further payoff would be new industries that can scale up enough to reduce costs of business, kind of like happened with wind and solar and batteries.
Two Bookends For The Reincarnation Of Hydrogen—ExxonMobil And Verdagy (forbes.com)